Fifty years after the first person walked on the moon, the relatively new field of planetary geology provides fresh insights into the origin of Earth, our solar system and the essence of life.
PROLOGUE
In the field of planetary geology, 1969 was a transformational year. In early February, a meteorite fell near the village of Pueblito de Allende in northern Mexico, scattering more than two tons of fusion-crusted stones over an area of 75 square miles. Five months later, Apollo 11 made the first moon landing and brought 22 kilograms (48.5 pounds) of lunar material back to Earth. Then, at the end of September, a meteorite blazed over the southern Australian town of Murchison, showering more than 100 kilograms of rocks containing amino acids essential for life.
At the time, these three events may not have seemed to have anything in common, but over the past 50 years the data collected from them and similar occurrences have helped scientists piece together answers to a cosmic puzzle. The questions they’ve pondered include: What processes led from the origin of the cosmos to the creation of our solar system? What caused the formation of the moon? And what makes Earth so special that we have life, which as far as we can tell seems to be unique in the universe?
The potential existence of extraterrestrial life has long fascinated scientists. During a lunch with colleagues at the Los Alamos National Laboratory in 1950, physicist Enrico Fermi famously asked, “Where are they?” Fermi, who had won the Nobel Prize in Physics in 1938, reasoned that some of the billions of stars in the Milky Way similar to our sun should have had planets capable of sustaining intelligent life. Furthermore, he posited, some of these life forms should have developed advanced technologies capable of interstellar travel. Given that many of these stars are billions of years old, there has been ample time for aliens to have visited our planet, yet there are no signs of extraterrestrial life — the Fermi paradox.
In 1961, astrophysicist Frank Drake tried to quantify the answer to the Fermi paradox as a series of conditional probabilities eventually leading to the detection of alien life. The Drake equation, which attempts to calculate the number of alien civilizations in the Milky Way capable of communicating with Earth, has been a core piece of the debate over the search for extraterrestrial intelligence (SETI). “A search of hundreds of thousands of stars in the hope of detecting one message would require remarkable dedication and would probably take several decades,” Drake wrote in a January 1997 Scientific American article co-authored with renowned astronomer Carl Sagan.1
More than two decades later, searching for a definitive answer to Fermi’s question, thousands of people around the world are using computers to monitor radio signals for signs of extraterrestrial intelligence through projects like SETI@home. Advances in planetary geology over the same period have provided a clearer picture of what special conditions were present in our solar system that generated the basis for life on Earth. This information may not answer the probability-of-extraterrestrials question, but it does help us appreciate what factors make us unique and hence better estimate the likelihood of similar life existing elsewhere in the universe.
To understand planetary geology, readers need to be familiar with some astronomical objects, as these are the actors in our story. Our solar system consists of the four inner terrestrial planets (Mercury, Venus, Earth and Mars), the main asteroid belt, the four ice giants (Jupiter, Saturn, Uranus and Neptune) and the Kuiper belt. The asteroid belt is the ring of rocks, rubble and other material orbiting the sun between Mars and Jupiter that, because of the velocities generated by the gravitational resonances with Jupiter, cannot coalesce into larger bodies. Collisions in the asteroid belt can lead to material falling toward the terrestrial bodies, causing meteorite impacts on Earth. Most meteorites are mainly bits of asteroids, but some come from Mars and our moon.
The Kuiper belt is a second outer ring, just beyond Neptune, and is much larger than the asteroid belt. While most asteroids are composed of rock and metal, the objects in the Kuiper belt are made up of ices, including methane, ammonia and water. Pluto, which astronomers demoted to dwarf planet status in 2006, is a member of the Kuiper belt. Comets (basically, cosmic snowballs) are former members of the Kuiper belt originating from the farthest region of our solar system, the Oort cloud.
The sun is truly the center of our world, containing 99.86 percent of our solar system’s mass. (Jupiter and Saturn comprise more than 90 percent of the rest.) Jupiter, the largest planet, is about five times farther from the sun than Earth is, and most of the planets are locked into orbital period resonances with it. (Saturn, for example, is in a 2:5 resonance with Jupiter, meaning it orbits the sun twice for every five of Jupiter’s orbits.)
But our solar system represents a tiny corner of the universe. The Milky Way, our galaxy, has roughly 100 billion stars, some larger and some smaller than our sun. The visible universe has about 10,000 galaxies in each of its three dimensions, or 1 trillion galaxies in total (10,0003).2
PART 1: THE MOON
On July 20, 1969, at 10:56 p.m. EDT, astronaut Neil Armstrong began climbing down the ladder of the Apollo 11 lunar module. Moments later, he proclaimed, “That’s one small step for a man, one giant leap for mankind.” Buzz Aldrin joined Armstrong on the moon’s surface 19 minutes later. I watched these historic footsteps on a black-and-white television with my brother and parents at home in Monterey, California. I was not alone: The first of six moon landings was estimated to have been watched live by 600 million people, nearly one-fifth of the world’s population at the time.
Fifty years later, the Apollo 11 lunar mission is still one of mankind’s greatest scientific and technical accomplishments. For me, like many others, the event inspired an interest in the sciences most clearly associated with the space program: physics and astronomy. Certainly, the space program deserves recognition for advancing those fields. But geology, the one science I did not appreciate at the time of the lunar landings, may be the most important science when it comes to understanding the formation of the moon and solar system. In fact, the only Ph.D.-level scientist in the astronaut program who walked on the moon, Apollo 17’s Harrison (Jack) Schmitt, was a geologist.
In 1969, the main scientific reason to visit the moon was to bring back lunar samples. If you read the literature or watch the many documentaries and recent movies about Apollo 11, you will notice that after Armstrong said his famous words and inspected the lunar module exterior for damage, he gathered contingency lunar samples and placed them in his collection bag in case an emergency ascent was required.3 If just this material had been recovered, the entire space program would have been a scientific success. Fortunately, the Apollo moon missions brought back significantly more, a total of 220 kilograms of rocks,4 providing samples from six sites chosen for their distinctive geological features.
Late Heavy Bombardment
The moon’s surface is actually fairly homogeneous. When we look at it with our naked eyes, the first thing that stands out are the lunar maria (Latin for “seas”), multiple large dark impact craters so deep that they once generated volcanic outflows. The maria are surrounded by the highlands, or terrae, bright areas created by meteorite impacts that did not penetrate the moon’s crust. Rocks called regolith breccias5 make up some 80 percent of the lunar highlands, which almost completely cover the far side of the moon.6 (Earth has regolith breccias as well, but they are not commonly seen due to the effects of weather and recycling of the surface.) Using fairly precise isotopic dating of the Apollo lunar samples, scientists were surprised to discover that the recovered breccias were created over a relatively narrow time period 3.9 billion to 4.0 billion years ago, during a period they called the Late Heavy Bombardment. Until 2018 it was accepted that nearly all of the visible craters on the moon were created during this period.7 However, recent analysis indicates that the formation of the moon’s craters may have occurred from 3.9 billion to 4.4 billion years ago; the narrow range indicated by the Apollo samples may be due to the relative closeness of the landing sites.
The Late Heavy Bombardment was a time of great turmoil and activity in the formation of our solar system. In fact, the asteroid bombardment affected Earth even more than the moon, but because of the recycling of Earth’s crust, it is not as obvious. Geologists have noticed for years that the rocks in the crust have an unusually large amount of iron-loving elements such as gold, palladium and platinum, which should have ended up in the planet’s core during the initial stages of its formation. These metals are believed to have come from a continuous process of asteroid and comet bombardment — in particular, the accretion of a late veneer on Earth’s surface roughly 3.5 billion to 4.5 billion years ago. The peak accumulations of the late veneer8 took place during the end of the Late Heavy Bombardment.9
The moon’s gravity is very weak — during the Apollo 11 mission, Aldrin and Armstrong bounced along the lunar surface as they set up experiments and collected rocks. As a result of this weak gravity, any major asteroid impact on the moon’s surface would generate projectiles with speeds greater than the moon’s escape velocity. Computer simulations indicate that most of these projectiles would be directly captured by Earth.
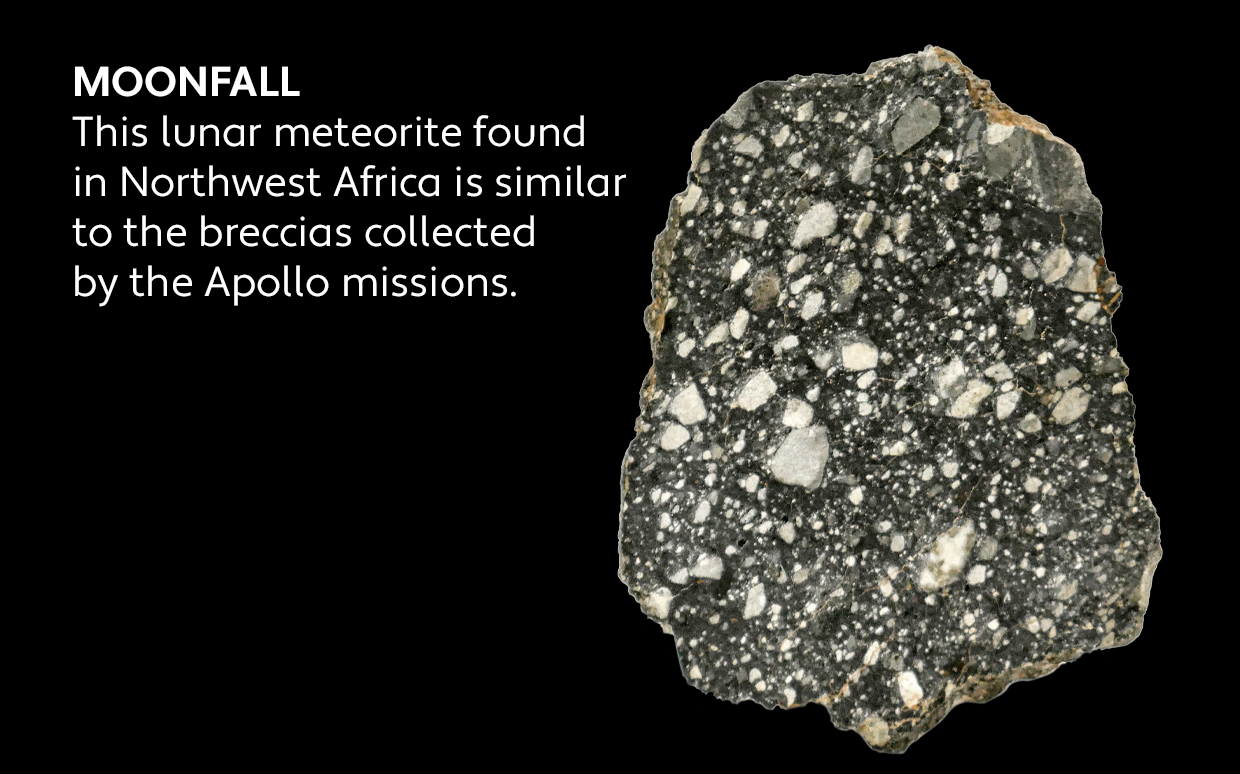
The first meteorite recognized as originating from the moon was identified during the Japan–U.S. Antarctic expedition of 1982 and confirmed by isotopic and chemical matching with Apollo lunar samples.10 (The surface of Antarctica’s ancient ice floes turns out to be an excellent place to find meteorites.) Cosmic ray exposure data, which measure the time meteorites traveled in space outside the protected atmosphere of Earth, indicate most of these lunar meteorites were ejected from the moon less than 20 million years ago. In total, there are now about 300 known lunar meteorite finds, representing approximately 30 distinct lunar impact events and totaling 190 kilograms of material.11 This is slightly less in total mass than the amount brought back by the Apollo astronauts, but it samples more distinct sites. The lunar meteorites found on Earth are randomly distributed samples of the lunar surface, including from the far side of the moon.12 They are entirely consistent with the lunar samples from the Apollo missions and have the same formation dates as the Apollo lunar samples – namely, the period of the Late Heavy Bombardment.
Lunar Origin Story
So let’s consider the origin of the moon. Was it captured by Earth or generated by some other process? Why does it exist at all? The answers to these questions can be found in the bulk properties of the lunar samples. When scientists compare the ratios of various elements in them with those of ordinary rocks on Earth, they find many exact matches, indicating that the moon and Earth share a common geological origin.13 It took until the mid-1980s for scientists to come up with enough additional data and analysis to put forth a narrative to solve this puzzle. They hypothesized that about 50 million years after the birth of the solar system, early Earth was struck by a Mars-sized planet, now called Theia.14
Theia may have initially formed at one of Earth’s two stable Lagrangian points. These are positions in space where an object can remain in orbit equidistant between two larger bodies — in this case, the sun and Earth. It is unclear what may have dislodged Theia from its orbit, as there are currently no known coincident events recorded in geological or meteorite history. Whatever the cause and wherever Theia initially orbited, the impact of Theia on Earth may have been violent enough to eject large parts of the original Earth’s mantle into space and vaporize the entire planet into what is called a synestia, a spinning torus of vaporized rock.15 In this scenario the moon would have cooled off faster and condensed first, followed by the new Earth.
PART 2: THE SOLAR SYSTEM
On February 24, 1969, in preparation for their lunar landing, Buzz Aldrin and Neil Armstrong went on a geology field trip near Sierra Blanca in West Texas.16 There they practiced collecting rock and soil samples among the ruins of an old U.S. Army garrison.
Two weeks earlier, the Allende meteorite had fallen about 400 miles south of Sierra Blanca, in the Mexican state of Chihuahua.17 The meteorite, estimated to be roughly the size of a car, had exploded as it entered Earth’s troposphere, raining down more than two tons of material for geologists to study. The timing was propitious, as many scientists were in the process of preparing their laboratories to analyze lunar rocks.
There are two types of meteorites: falls and finds. Falls represent observed events. They are very dramatic, as meteorites like Allende enter Earth’s atmosphere at speeds typically greater than 11 kilometers per second (25,000 mph), causing bright streaks, flashes and glass-breaking sonic booms as they deaccelerate due to atmospheric friction. Such events occur multiple times per day, but fewer than 20 per year are recovered by meteorite hunters and made available for scientists to analyze, as well as for museums and private collectors.
Finds are discoveries of previous falls — some ancient — and are typically discovered in deserts or on top of ice fields due to the ease of detection. Finds provide us with a record of meteorite falls over eons and can often be dated based on the strata in which they are discovered. As meteorite science is only a few generations old, there are presently more finds than recovered falls. And ultimately, the accumulated mass of finds blends into the continuum of what we call the planet’s soil and crust; meteorites represent at least 0.5 percent of Earth’s total mass.
Allende is one of the most primitive types of meteorites, known as carbonaceous chondrites because they’re rich in carbon. Like most meteorites, the Allende meteorite has a dark fusion crust generated during its flight through Earth’s atmosphere; the inside is a dark gray and white grainy material. The meteorite has three distinctive features: small pebbles known as chondrules, a dark background called the matrix and an extended white material known a refractory inclusions. The inclusions are bright white and consist of calcium and aluminum, so they are also known as calcium-aluminum-rich inclusions (CAIs).
Solar System Origin Story
What caused our solar system to form in the first place? The Allende meteorite provides key insights that help answer that question. In 1977, a group of scientists from the California Institute of Technology reported that Allende showed signs of a magnesium crystal compound that formed when the magnesium was originally aluminum-26.18 This element has a relatively short 700,000-year half-life and is produced by what are known as Type II supernovas — dramatic explosions of large stars with masses ranging from eight to 50 times that of our sun. That same year, Harvard University astrophysicist Alastair G.W. Cameron published a paper with longtime University of Chicago professor James Truran making the case that a Type II supernova triggered the collapse of a giant molecular gas cloud to begin the formation of our solar system.19
The refractory inclusions in the Allende meteorite are enriched with products of that supernova, and the decay process of those supernova-based elements in Allende allows scientists to date its formation, and hence the supernova, with surprising accuracy. Refractory inclusions were some of the first matter to form around the early sun. Allende marks it as 4.567 billion years ago, plus or minus 1 million years (accurate to four significant figures).20 This is about 30 million years before Earth’s formation.
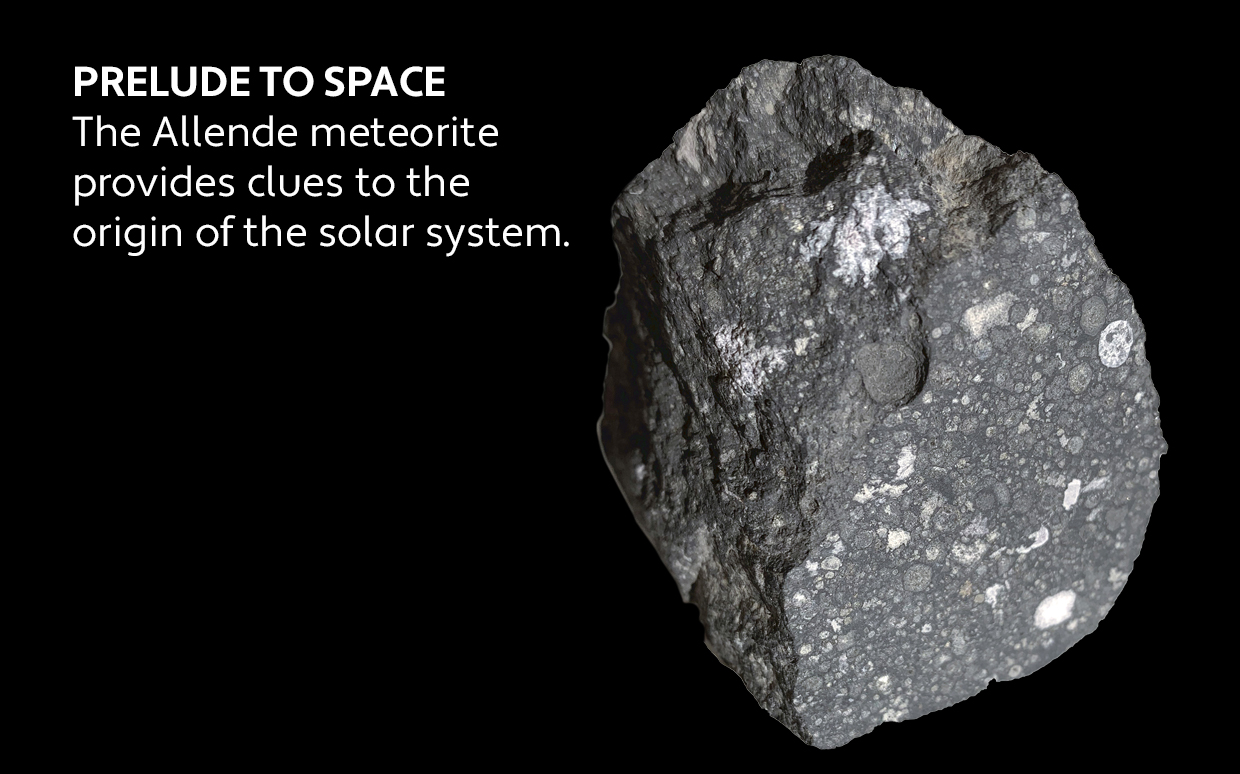
Chondrules and matrix in the Allende meteorite better represent the other main source of material — a giant molecular cloud. The chondules were produced by flash heating to about 1,000 degrees Kelvin (1,340 degrees Fahrenheit) during early interactions of the cloud material as it was swept into and processed by the new sun and subsequently mixed into the planetary disk along with the CAI. This phenomenon was described in detail by X wind theory, proposed in 1996 by University of California, Berkeley, astronomer Frank Shu.21 However, this is still a very active research area, and more advanced models can now better satisfy the chondrule and CAI distributions found in meteorite data.
Chondrules occur in most meteorites. As millimeter-sized objects, they coagulated with the cloud’s dust grains to help form at least 100 planetary seeds. These seeds eventually became the major elements of our present solar system. From start to finish, the collapse of a fragment of a giant molecular cloud to create the sun and solar system took about 60 million years.
The Kilonova Event and Stardust
On August 17, 2017, scientists at the Laser Interferometer Gravitational-Wave Observatory (LIGO) detected the first kilonova as two neutron stars in the Hydra constellation, 130 million light-years from Earth, collided.22 The explosion set off fireworks across the universe; spectral analysis revealed heavy element signatures in the trailing cloud of dust. Scientists estimate that the kilonova could have produced a quantity of gold more than 40 to 100 times Earth’s mass.
In May 2019, scientists observed that Allende carries excess heavy elements in relationship to the data gathered by LIGO, consistent with a similar neutron star event about 80 million years before the supernova.23 A colliding neutron star event generates a thousand times more gold than a Type II supernova; this may explain Earth’s excess of so-called r-process metallic elements, such as gold, silver, platinum, plutonium and uranium.
In fact, many other stars contributed material to the giant molecular cloud that condensed to form our solar system. Primitive meteorites such as Allende and Murchison contain microscopic presolar grains, known as stardust, which are the end product of earlier generations of stars. Many grains are dated to have formed around 7 billion years ago (about half the age of the universe), indicating an intense period of star formation and activity in the Milky Way during that time.
Giant Planet Migration
Although the sun, Earth, moon and planets formed over a period of about 50 million years, the stability of the system was not assured, because of remaining nebular material that continued to accrete into the planets as they cleared their orbits over a much longer timescale. Over time, the planets increased in size, causing a realignment of the “music of the spheres,” defined as the multibody synchronization of planetary resonances that stabilizes the solar system by preventing planets from approaching one another too closely. One of these realignments, called the giant planet migration, was a major event, second in importance only to the initial formation of the solar system, and occurred some 100 million to 560 million years after the initial system formation, or as late as 4 billion years ago, according to most experts.
Giant planet migration is supported by the Nice computational hydrodynamic model, now widely accepted as the standard scenario for the evolution of the solar system. In the Nice model — initially developed in 2005 by scientists Rodney Gomes, Harold Levison, Alessandro Morbidelli and Kleomenis Tsiganis while working together at the Côte d’Azur Observatory in Nice, France — Neptune’s orbital drift caused a flux of the icy Kuiper objects inward, toward the center of the solar system. Jupiter, whose increasing mass was a major source of Neptune’s instability, scattered many of the incoming Kuiper objects, and as it lost angular momentum it moved inward. At some point, its orbital resonance with Saturn passed through the 1:2 ratio, temporarily increasing Saturn’s orbital eccentricity and pushing Neptune out farther. Via this feedback loop, most of the original Kuiper belt was scattered, either inward and potentially into unstable planet-crossing orbits or outward to what is called the scattered disk, where the comets now reside. These shifts in Jupiter’s orbit also perturbed the asteroid belt, causing many asteroids to drift into planet-crossing orbits. The Nice simulations indicate that this process was a fairly rapid phase transition compared with the lifetime of the solar system. This is how the giant planet migration caused the Late Heavy Bombardment.
Part 3: LIFE ON EARTH
On April 23, 2019, at 9:07 p.m., residents of the town of Aguas Zarcas in Costa Rica saw a fireball streaking across the sky. The meteorite, about the size of a washing machine, broke apart during its descent, with some of the larger fragments crashing into occupied buildings. One piece smashed through the roof of a doghouse. Fortunately, no people or animals were hurt.
Over the next few days, meteorite hunters descended on Costa Rica in a race to find or purchase the fragile rocks that had fallen. Meteorite hunters — including Michael Farmer, Bruno Fectay and Carine Bidaut, Robert Haag, Siegfried Haberer, Greg Hupe, Viacheslav Kalachev, Achim and Moritz Karl, Luc Lebenne, Geoff Notkin and Steve Arnold, and Robert Ward — specialize in the logistically challenging and sometimes dangerous work of rapidly recovering both falls and newly discovered finds. They are modern-day Indiana Joneses, combining adventure, detective work and scientific techniques to preserve the valuable samples for future generations.24 Because the Aguas Zarcas meteorite25 is a rare CM-type chondrite composed of clay, it was important to recover samples before it could be broken down and contaminated by rain. The Aguas Zarcas meteorite has a distinctive musty odor from extraterrestrial organic compounds, validating its origin in a carbonaceous asteroid.
Using astronomical observations from as far away as Brazil, astronomers were able to reconstruct the orbit of the Aguas Zarcas meteorite; as expected, it originated in the outer asteroid belt. From the spectral analysis of flyby missions, the parent body of CM meteorites is likely to be the 19 Fortuna asteroid (the 19th asteroid discovered), which has a diameter of 200 kilometers, making it one of the largest bodies in the asteroid belt.26 The CM meteorites are relatively fragile in space; hence, it is believed that Aguas Zarcas was dislodged from Fortuna’s surface less than 1 million years ago.
Aguas Zarcas has drawn comparisons to another CM meteorite, which fell near the small riverside village of Murchison in Victoria, Australia, on September 28, 1969 — like Allende, it is another well-studied meteorite. Whole stones of the Murchison fall were recovered largely intact, with the inner portions of material essentially free of any contamination from terrestrial sources. Yet CM meteorites like Murchison and Aguas Zarcas contain significant amino acids, phosphoric acids, sugars and alcohols, as well as meaningful quantities of aromatic hydrocarbons.27 These compounds are clearly organic in nature but not yet alive or generated by life, so we call them prebiotic organic compounds.
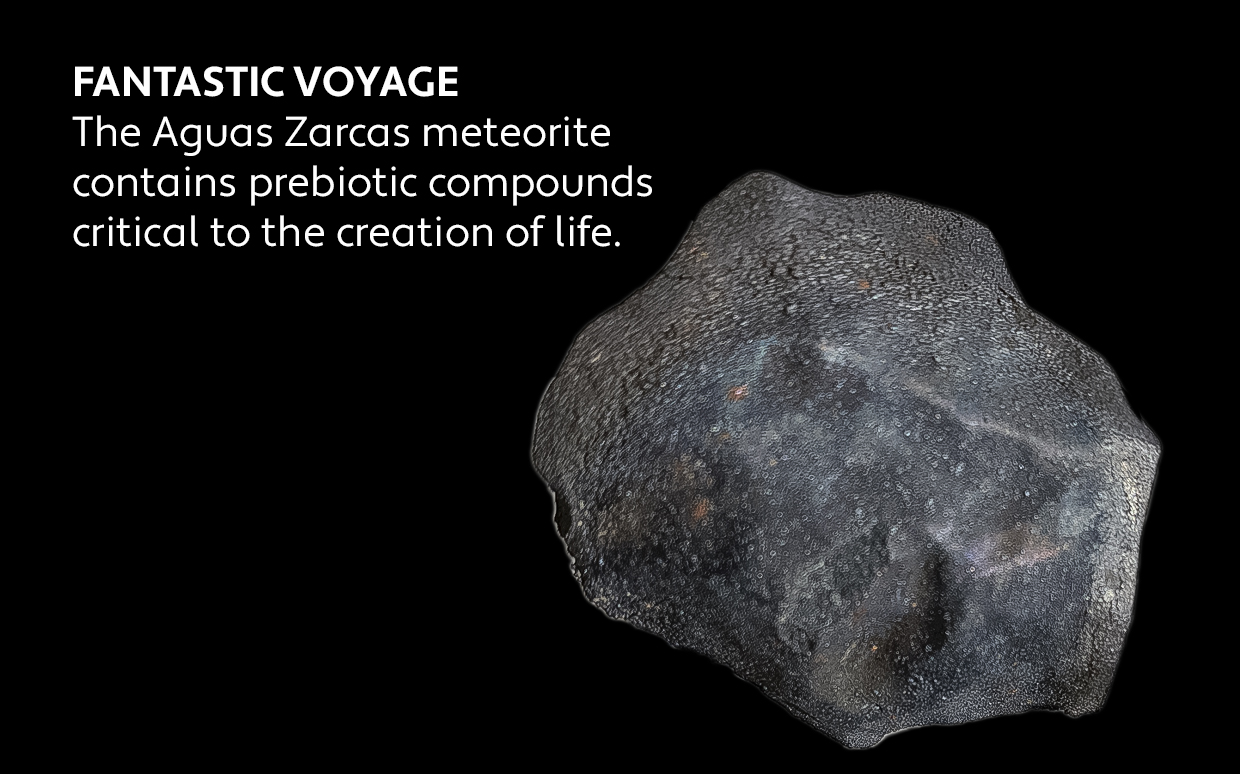
Amino acids are chiral, meaning they come in two configurations — left-handed and right-handed — that are mirror images of each other. The amino acids found in the CM meteorites are largely left-handed. Life on Earth, of course, is based almost exclusively on left-handed amino acids.28 All this is consistent with the notion that the organic compounds on Earth that produced the advent of life and the prebiotic compounds in the meteorites have a common origin. In particular, if the sun formed close to a cluster of massive stars, polarized radiation from those stars may have more preferentially destroyed the right-handed versions of the organic molecules. Observations indicate that polarization is a near-universal feature of star-forming regions.29
The Cosmic Carbon Pathway
Where did these prebiotic organic compounds arise? It turns out they originated in the universe’s first generation of stars — in particular, the carbon stars, red giant stars that have more carbon than oxygen in their atmospheres. In these stars at the end of their stellar evolution, the elements oxygen and carbon combine to form carbon monoxide until the oxygen is consumed. At this point, the carbon is free to form other compounds, giving the star a sooty atmosphere. As the star shreds itself in the final stages of its life, this matter accumulates to form dense clouds in the interstellar medium (ISM). The outflow from these late-stage stars includes such complex molecules as polycyclic aromatic hydrocarbons (PAHs), as found in the CM meteorites, and small carbon and silicate dust particles. These compounds are modified in the ISM through various physical and chemical processes.30 In the ISM, nearly all free oxygen combines with hydrogen to form water; with the temperature ranging between 10 and 50 degrees Kelvin (–442 to –370 degrees Fahrenheit), most of this water is in ice form and accretes on carbon and silicate grains.31 This forms the giant molecular clouds.
Using the Spitzer infrared space telescope, scientists have discovered that giant molecular clouds are found throughout most galaxies. In our nearby sister galaxy, Andromeda, they form a density distribution coincident with the galaxy’s spiral arms when viewed in infrared. So we now understand that the cosmic carbon pathway to Earth begins with the formation and evolution of giant molecular clouds.
These clouds became the nurseries of the second and third generations of stars. In our galaxy, which has a total mass of 2 billion solar masses, the rate of star formation from clouds is approximately one per year. This is currently in equilibrium with the gas ejected by the red giants, making the amount of giant molecular clouds in the Milky Way relatively stable. It is this material that blocks our view of the central part of our galaxy. Looking up at the Milky Way, you can perceive the clouds as dark patches, backlit by the glow of the galaxy’s stars.
Our own solar nebula originated from a giant molecular cloud. The best representation of this original cloud material in our current solar system are the comets and carbonaceous asteroids. The environment of the larger asteroids during the formation of the solar system may have also been suitable for further chemical processing of their accumulated prebiotic materials.
The second part of the cosmic carbon pathway has taken place continuously since the Earth cooled after the Theia impact, accomplished by the delivery of material to Earth in the form of meteors and meteorites.32 Via this mechanism, the asteroids and comets have provided over time much of the water33 that has formed the oceans on Earth.34 Asteroids have a similar ratio of deuterium (heavy water) to regular water as that found on Earth, while comets have a much higher ratio, as suggested by the European Space Agency’s Rosetta mission.35 The data analysis from this mission suggests that the water provided to Earth came mainly from asteroids, not comets.36 Carbonaceous asteroids are from the outer asteroid belt closer to Jupiter and beyond the frost line, where frozen water can exist without being vaporized by the solar wind. These asteroids contain significant amounts of ice, typically exceeding 10 percent of their mass.
The prebiotic organic molecules on Earth were contributed by atmospheric synthesis, comets, asteroids and interplanetary dust. Carbonaceous meteorites also have similar relative abundances of amino acids created in the famous Miller–Urey experiment, first conducted in 1953 by American chemists Stanley Miller and Harold Urey to simulate the synthesis of organic compounds from methane, ammonia, hydrogen and water vapor in Earth’s early atmosphere.37
Carl Sagan and Christopher Chyba, an astrobiologist at Princeton University, initially estimated the flow of extraterrestrial organics to Earth in 1992, based on lunar cratering data.38 Scientists currently believe that meteorites contributed at least 1 billion kilograms of organic carbon per year during the Late Heavy Bombardment, five times greater than what was being produced in Earth’s atmosphere from the Miller–Urey mechanism.
The Genesis of Life
Life on Earth began about 3.9 billion to 4.1 billion years ago, at the end of the Late Heavy Bombardment, with one branch reflected in our shared ancestry as a single-cell organism known as the last universal common ancestor (LUCA). This organism relied on synthesized organic molecules from the prebiotic soup. In 2016, American evolutionary biologist William Martin led a team of researchers that found conclusive genetic evidence that LUCA originated near anaerobic thermal vents in geochemically active environments in the prebiotic oceans,39 challenging the then-prevailing theory that life on Earth began in land-based pools. The genetic evidence specifically indicates that LUCA used a set of biochemical reactions called the Wood–Ljungdahl pathway in a hydrothermal vent. LUCA fixes carbon from the environment by converting carbon dioxide into carbon monoxide using metals available in the water with a temperature between 86 and 212 degrees Fahrenheit and without the need for proteins.
The genesis of life may have benefited from the fact that Earth’s outer shell is divided into light plates, which move over a dense mantle. While the precise start date of Earth’s plate tectonics is still vigorously debated, a preliminary version of it could have provided early life with a consistent, hydrothermal environment, as ocean water filtered down through the crust into the mantle at plate boundaries and was subsequently reinjected at suboceanic ridges at high temperatures. This process would have turned Earth into a giant incubator, with thousands of miles of thermal vents bubbling away, pumping methane, carbon dioxide, metals and hydrogen into an ocean of cooler prebiotic organic compounds. At some point during the incubation process, there was a LUCA metabolic event40 in a hydrothermal vent. According to Martin, the porous iron sulfide 3D structures that formed in the vents served as primitive cell walls and a stable environment for the eventual creation of the RNA molecule ribozyme from the existing prebiotic compounds — providing the genesis of life’s replicating mechanism.
Other Life in the Universe
Looking beyond Earth, the conditional probabilities set forth in the Drake equation provide a framework for analyzing the amount of life in the universe.41 However, the estimates of the combined probabilities are overwhelmed by the uncertainty associated with the derived values, making it impossible to come to firm conclusions. Still, we can now endeavor to improve the accuracy by examining the local planetary systems for candidates that might have had thermally activated prebiotic compounds in an aqueous setting.
In our solar system, we have at least four such candidates: Jupiter’s moons Europa and Ganymede, Saturn’s moon Enceladus and the planet Mars. Europa has plate tectonics of surface ice crust and is geologically active, and Enceladus and Ganymede both indicate the existence of volcanic activity in their subsurface oceans. There are active scientific programs to study all three: the NASA Europa Clipper, the German Aerospace Center Enceladus Explorer and the Russian Space Research Institute Ganymede Lander mission.
In the case of Mars, we have much more information. Geologically, the planet’s most prominent feature is the striking difference between the northern lowlands and southern highlands on its surface, known as the Martian dichotomy. This change in the thickness of Mars’s crust runs along an apparently irregular boundary between its northern and southern hemispheres. Scientists believe that the elliptical nature of this boundary was caused by the impact of a giant body (again during the Late Heavy Bombardment), creating the largest such impact structure thus far identified in the solar system and initiating a period of plate tectonics.42
Supplementary evidence indicates that Mars consists only of these two plates, north and south.43 The northern depression was actually filled with water for a significant period, just like Earth’s oceans.44 The conditions on Mars may have been remarkably similar to Earth’s for some time period; this also suggests that water-based plate tectonics may not be a rare event. Unfortunately, during the end of the Late Heavy Bombardment, one collision disrupted Mars’s magnetic field, significantly reducing protection from the solar wind and causing the ocean to evaporate and most of the ancient atmosphere to dissipate into space.45 At present, the location of water across the planet now seems to be mainly below the surface in the form of ice. Mars is still, however, occasionally active geologically, and with significant remaining surface ice at the polar regions, likely pockets of subsurface water and an atmosphere that is 96 percent carbon dioxide, the planet is worthy of further examination for life.
EPILOGUE
As with the Copernican revolution, in which the sun replaced Earth as the center of the universe nearly 500 years ago, we now know that Earth itself is not at the center of the creation of materials essential for life. The organic compounds that seeded the early oceans have a mainly extraterrestrial origin via the cosmic carbon pathway, and they are observable as giant molecular clouds in galaxies throughout the universe. Circular radiation from larger nearby stars, or the supernova that collapsed the cloud, may have preferentially destroyed the right-handed polarized amino acids in our solar system, leading to chiral organics.
The delivery pathway of this material from the heavens to Earth is still robust and continues to this day in the form of meteorites and cosmic dust. Many of the ancient planetary seeds that formed the early solar system were transported via this pathway and exist on Earth in the form of iron meteorite finds — hence our planet’s geological record contains other worlds to study. Meteorites have proved invaluable to unraveling the cosmic puzzles of the origin of both the solar system and life.
During the space race in the 1960s and ’70s, the Soviet Union and the U.S. launched multiple spacecraft intended to fly by Mars. NASA’s Mariner 4 was the first to reach the Red Planet, on July 14, 1965, sending 21 close-up photos back to Earth.46 NASA’s Viking mission,47 which landed two unmanned spacecraft on Mars in 1976, measured the chemical composition of the planet’s soil and atmosphere.48 Based on this data and other factors, scientists concluded in 1983 that Shergottite meteorites, an igneous rock that had been unclassified for almost 100 years, were of Martian origin. One of the multiple factors pointing to this conclusion is that the gases trapped in a glassy portion of Shergottite meteorites match the ratio of elements in the Martian atmosphere measured by the Viking landers.
There are now more than 100 known finds of Martian meteorites; the total amount of Mars on Earth is more than 100 kilograms.49 However, there are only five observed falls of Martian meteorites, which, interestingly, occur roughly once every 50 years. The last Martian meteorite was a Shergottite called Tissint that fell on July 18, 2011, close to the southern Moroccan town of the same name.50 Tissint meteorites contain trapped carbon-based organic compounds. These compounds were originally thought to be possible markers of life, but today scientists believe they originated from volcanic activity combined with aqueous alteration of the rock material; this is consistent with the theory that there is water on Mars. There is more evidence of water on Mars, 2.5 billion years ago, from the Northwest Africa 7034 meteorite — dubbed “Black Beauty” — found in the Sahara desert in 2011. The oldest known Martian meteorite, Allan Hills 84001, discovered in Antarctica in 1984, shows that liquid water existed on the surface of Mars as far back as 4 billion years ago. All of this is consistent with the delivery of water to Mars by the same pathway that delivered water to Earth.
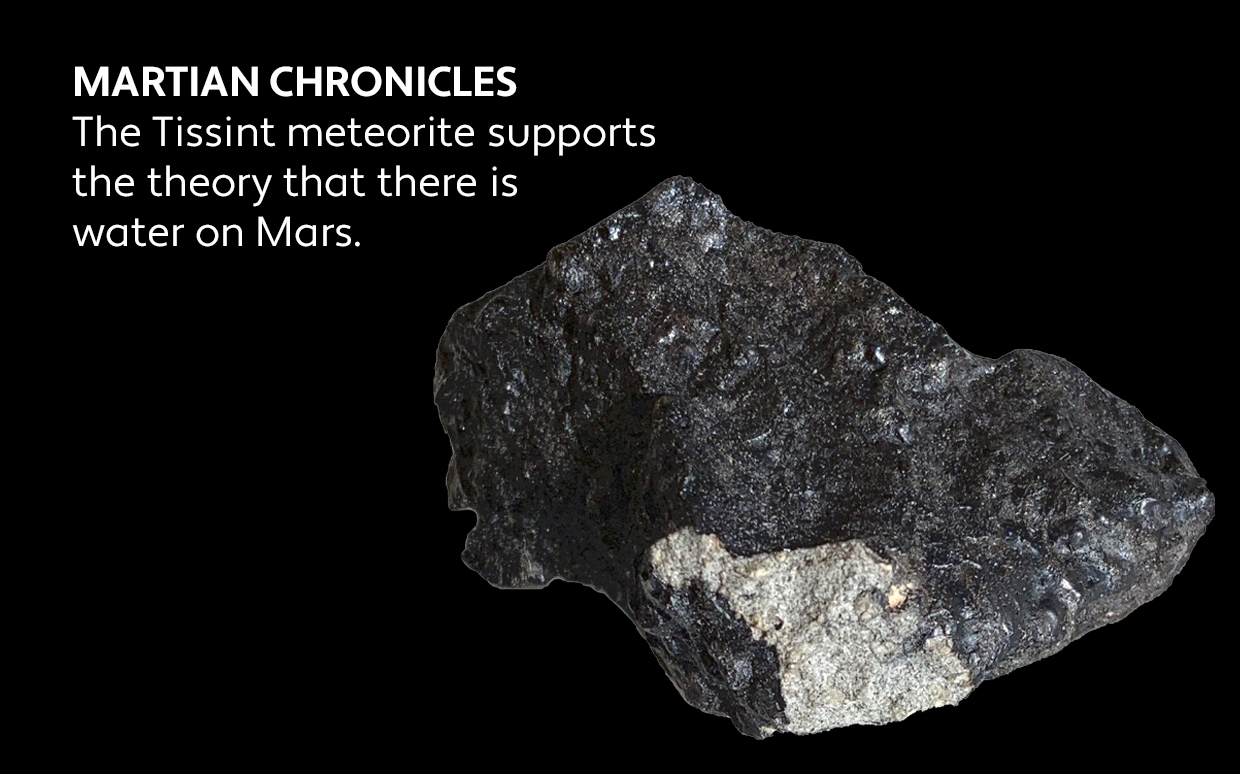
Mankind has long been fascinated with Mars, both because of its proximity to Earth and because of the possibility that it could support life. British astronomer Sir William Herschel believed that the dark areas on Mars were oceans; in 1784, he wrote that Martian inhabitants “probably enjoy a situation similar to our own.”51 In 1877, Italian astronomer Giovanni Schiaparelli began mapping Mars; he called the channels he saw canali, which was later mistranslated into English as “canals.”52 Two decades later, Percival Lowell, a wealthy American businessman and astronomer, published his first of several books on Mars, popularizing the idea that Martians built the canals to transport water from their polar ice caps. We now know that because of its thin atmosphere Mars supports surface water only in the form of ice, mainly close to its poles. However, empty canals formed by surface water in ancient times exist on Mars, and the situation that brought water to Mars was similar to Earth’s, so, ironically, there is truth to Schiaparelli’s and Lowell’s early conjectures.
Mars has been a popular subject for science fiction writers. In 1898, British author H.G. Wells published The War of the Worlds, a fictionalized first-person account of a Martian invasion of southern England. Growing up, I enjoyed reading stories about colonizing Mars by authors like Ray Bradbury and Arthur C. Clarke. In 2011, Andy Weir published The Martian, a novel about an American astronaut who is stranded alone on Mars and how he survives while waiting to be rescued.
Today a modern-day race to colonize space is playing out among private companies like Elon Musk’s SpaceX, Jeff Bezos’s Blue Origin and Richard Branson’s Virgin Galactic, and public entities including NASA, the Russian Federal Space Agency and the China National Space Administration. Musk, who founded SpaceX in 2002 to revolutionize space travel, may have the most ambitious goals: His company plans to transport humans to Mars by 2024, two years after sending its first unmanned cargo ship to the Red Planet “to put in place initial power, mining and life support infrastructure.”53 In a March 2019 tweet, Musk wrote that it will be possible to build “a self-sustaining city” on Mars by 2050.
Bezos, for his part, believes that the fastest way to get to Mars is by first establishing an outpost on the moon. “If you’re gonna need a lot of supplies and fuel and bulk materials to go to Mars, you’re much better off lifting them off the moon than you are lifting them off the Earth,” Bezos said in a June 2019 interview, at an event to celebrate the 50th anniversary of the lunar landing.54
Hopefully, these human space adventures will include some geologists. As billionaires like Musk, Bezos and Branson continue to push human exploration beyond the bounds of Earth, and as public agencies and their scientists continue to make new discoveries via robotic missions, we may someday accumulate enough data and insights to solve the Fermi paradox.
So what have we learned over the past 50 years when it comes to the probability of extraterrestrial life?
Well, we now understand that underwater geothermal activity provided the incubation mechanism for a primordial soup delivered by a continuous bombardment of water and organic material that was molecularly chiral onto a relatively cool planet surface. Although there are specific “Goldilocks” parameters for Earth’s special case, this scenario itself may not be extremely unusual for solar systems.
The initial organic materials are available throughout the universe in the form of giant molecular clouds produced by first-generation stars, so planets only need to be capable of the incubation process; they do not have to create organic chemistry from scratch. Therefore, it seems that for now the Fermi paradox is as confounding as ever — possibly even more so. In this context, there is significant value in understanding the other candidates in our own solar system capable of life to refine the criteria and better appreciate what makes our lovely planet so special.
Paul Griffin is Chief Science Officer of WorldQuant. He collects meteorites and has a PhD from Stanford University and BA from the University of California, Berkeley, both in physics.